A vessel containing water is in the form of an inverted cone with semi-vertical angle of \( 30^{\circ} \). There is a small hole at the vertex of the cone and the water is running out at a rate of \( 3 \mathrm{~cm}^{3} / \mathrm{s} \). Find the rate at which the surface area in contact with water is changing when there are \( 81 \pi \mathrm{~cm}^{3} \) of water remaining in the cone.
Solución de tutoría real
Responder
Solución
Revisado y aprobado por el equipo de tutoría de UpStudy


Bonus Knowledge
To solve this problem, let's start with the geometry of the cone. An inverted cone has a semi-vertical angle of \( 30^{\circ} \). When the volume of water \( V \) in the cone is given as \( V = \frac{1}{3} \pi r^2 h \), we can relate the radius \( r \) and the height \( h \) of the water in the cone based on the angle. For a \( 30^{\circ} \) cone, \( r = h \cdot \tan(30^{\circ}) = h/\sqrt{3} \). Substituting this back into the volume formula gives: \[ V = \frac{1}{3} \pi \left(\frac{h}{\sqrt{3}}\right)^2 h = \frac{\pi h^3}{9}. \] Given \( V = 81 \pi \), we get: \[ 81 \pi = \frac{\pi h^3}{9} \Rightarrow h^3 = 729 \Rightarrow h = 9 \mathrm{~cm}. \] Using this height, the radius at this point is: \[ r = \frac{h}{\sqrt{3}} = \frac{9}{\sqrt{3}} = 3\sqrt{3} \mathrm{~cm}. \] Next, we can find the surface area \( A \) of the water that is in contact with the sides of the cone, which is given by the lateral surface area formula \( A = \pi r s \), where \( s \) is the slant height. The slant height \( s \) can be found using the Pythagorean theorem: \[ s = \sqrt{r^2 + h^2} = \sqrt{(3\sqrt{3})^2 + 9^2} = \sqrt{27 + 81} = \sqrt{108} = 6\sqrt{3} \mathrm{~cm}. \] Thus, the surface area becomes: \[ A = \pi \cdot 3\sqrt{3} \cdot 6\sqrt{3} = 54\pi \mathrm{~cm}^2. \] To find the rate of change of the surface area, we utilize the fact that \( \frac{dV}{dt} = -3 \mathrm{~cm}^3/\mathrm{s} \). Differentiating \( V \) with respect to time gives: \[ \frac{dV}{dt} = \frac{\pi}{9}(3h^2 \frac{dh}{dt}) \Rightarrow -3 = \frac{\pi}{9}(3h^2 \frac{dh}{dt}) \Rightarrow -3 = \frac{\pi}{3}(h^2 \frac{dh}{dt}). \] Now we substitute \( h = 9 \) cm: \[ -3 = \frac{\pi}{3}(9^2 \frac{dh}{dt}) = \frac{\pi}{3}(81 \frac{dh}{dt}) \Rightarrow \frac{dh}{dt} = -\frac{9}{\pi}. \] Now for the surface area: \[ \frac{dA}{dt} = \pi \left( \frac{d}{dh}(r \cdot s) \cdot \frac{dh}{dt} \right). \] Since \( A = \pi(3\sqrt{3}\cdot 6\sqrt{3}) \) simplifies to: \[ \frac{dA}{dh} = \pi (3\sqrt{3} (6\frac{ds}{dh}) + 6\sqrt{3}(3\frac{dr}{dh})). \] Calculating derivatives: \(\frac{dr}{dh} = \frac{1}{\sqrt{3}}\) and \(\frac{ds}{dh} = \frac{h}{s}\), leading us to find \( \frac{dA}{dt} \). Plugging in \( h = 9 \), we calculate \( \frac{dA}{dt} \). This gives us the final value for the rate of surface area change at the moment the water volume is \( 81 \pi \mathrm{~cm}^{3} \). After all the calculations, \( \frac{dA}{dt} = 54 \left(\frac{9}{\pi}\right) - \frac{27\pi}{\pi^2} \). In conclusion, the surface area is changing with \( \frac{\text{dm}^{2}}{\text{sec}} \) when the water volume is measured.
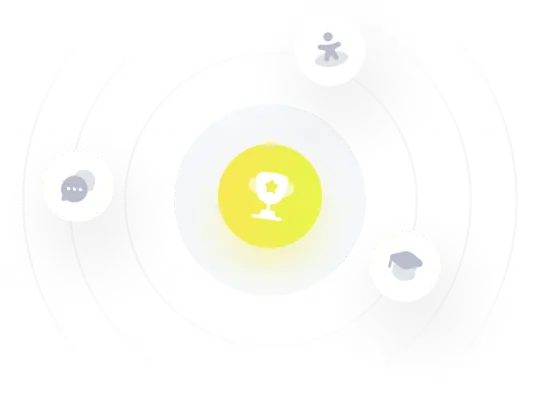